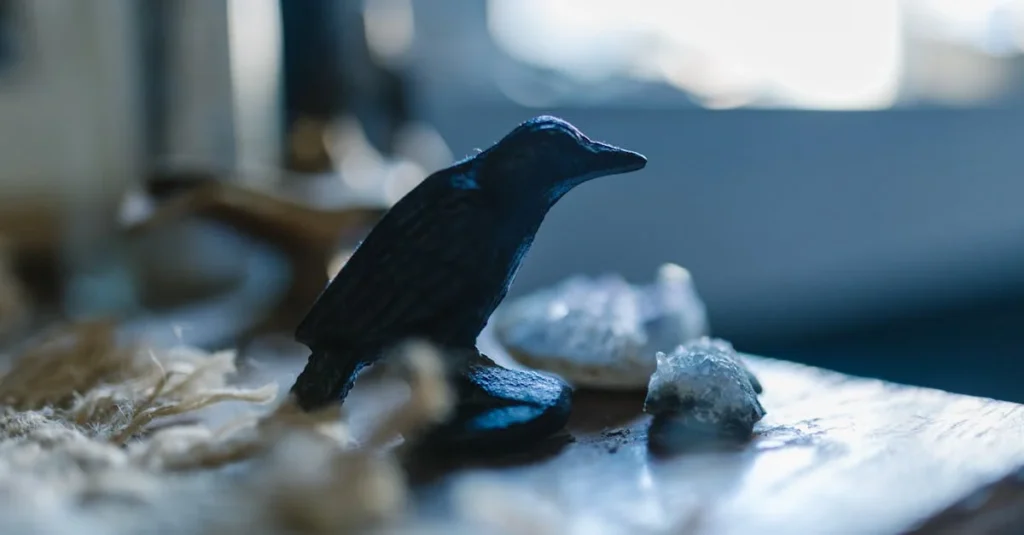
The periodic table of elements is a cornerstone of chemistry, organizing all known elements in a systematic way. As a chemist, I’ve always been fascinated by its elegant structure and the wealth of information it contains. This powerful tool not only arranges elements by atomic number but also reveals patterns in their properties and behavior.
From hydrogen to oganesson, the periodic table showcases the building blocks of our universe. It’s an indispensable resource for students, researchers, and professionals alike. In this article, I’ll dive into the intricacies of the full periodic table, exploring its history, organization, and practical applications. Whether you’re a chemistry enthusiast or simply curious about the world around us, understanding this fundamental scientific chart is key to unlocking the secrets of matter itself.
Key Takeaways
- The periodic table organizes all known elements by atomic number, revealing patterns in their properties and behavior
- Key components include atomic number, symbol, element name, and categories (metals, nonmetals, metalloids)
- Elements are arranged in groups (columns) and periods (rows), with main group elements, transition metals, lanthanides, and actinides
- Important trends include atomic size, electronegativity, and ionization energy, which vary across the table
- The periodic table has practical applications in chemistry, material science, education, and research
- Ongoing research focuses on synthesizing new superheavy elements and exploring the “island of stability”
Full:_qptvzwi-mg= Periodic Table of Elements
The periodic table of elements is a cornerstone of chemistry, providing a systematic arrangement of all known chemical elements. I’ll explore its history, development, structure, and organization to deepen our understanding of this fundamental scientific tool.
History and Development
The periodic table’s roots trace back to 1869 when Russian chemist Dmitri Mendeleev first proposed a systematic arrangement of elements. Mendeleev’s breakthrough came from organizing elements by atomic weight and observed chemical properties. He left gaps for undiscovered elements, accurately predicting their properties. In 1913, Henry Moseley refined the table by using atomic numbers instead of atomic weights, solidifying its current structure. Notable milestones include:
- 1829: Johann Wolfgang Döbereiner identifies triads of elements with similar properties
- 1862: Alexandre-Emile Béguyer de Chancourtois creates the first geometric representation of elements
- 1864: John Newlands proposes the Law of Octaves, noting patterns in element properties
- 1869: Dmitri Mendeleev publishes his first periodic table
- 1913: Henry Moseley establishes the use of atomic numbers for element arrangement
- 2016: IUPAC completes the seventh row of the periodic table with the addition of four new elements
Structure and Organization
The periodic table’s structure efficiently conveys crucial information about each element. Key features include:
- Rows (Periods): 7 horizontal rows, each representing an energy level or shell
- Columns (Groups): 18 vertical columns, grouping elements with similar properties
- Atomic Number: Unique identifier for each element, displayed at the top of each cell
- Symbol: One or two-letter abbreviation for the element’s name
- Atomic Mass: Average mass of an atom, shown at the bottom of each cell
Elements are organized into several categories:
- Metals: Left and center of the table, typically shiny and good conductors
- Nonmetals: Right side of the table, often brittle and poor conductors
- Metalloids: Elements with properties of both metals and nonmetals, found along the metal-nonmetal divide
- Noble Gases: Group 18, extremely stable and nonreactive
- Halogens: Group 17, highly reactive nonmetals
- Alkali Metals: Group 1, highly reactive metals
- Alkaline Earth Metals: Group 2, less reactive than alkali metals but still highly reactive
The table’s layout reveals periodic trends in element properties, such as:
- Atomic Radius: Decreases from left to right across a period, increases down a group
- Electronegativity: Increases from left to right across a period, decreases down a group
- Ionization Energy: Increases from left to right across a period, decreases down a group
By understanding these structural elements and organizational principles, I gain deeper insights into the relationships between elements and their behavior in chemical reactions.
Key Components of the Periodic Table
The periodic table is composed of several essential elements that provide crucial information about each chemical element. I’ll break down the key components that make this scientific tool so valuable and informative.
Atomic Number and Symbol
The atomic number, displayed prominently in each element’s box, represents the number of protons in an atom’s nucleus. It’s a unique identifier for each element, determining its position on the table. Alongside the atomic number, you’ll find the element’s symbol – a one or two-letter abbreviation that serves as a universal shorthand. For example, ‘H’ for hydrogen, ‘O’ for oxygen, and ‘Fe’ for iron. These symbols are consistent across languages, making them essential for international scientific communication.
Element Names and Categories
Each element box contains the full name of the element, providing immediate identification. The periodic table organizes elements into distinct categories based on their properties:
- Metals: Occupy the left and center of the table, typically shiny and good conductors of heat and electricity
- Nonmetals: Found on the right side, generally poor conductors with diverse properties
- Metalloids: Located along the diagonal line between metals and nonmetals, exhibiting properties of both
- Noble gases: The rightmost column, known for their stability and low reactivity
- Halogens: The second-rightmost column, highly reactive nonmetals
- Alkali metals: The leftmost column (excluding hydrogen), soft and highly reactive metals
- Alkaline earth metals: The second column from the left, reactive but less so than alkali metals
These categories help scientists predict element behavior and properties, streamlining research and experimentation processes.
Elemental Groups and Periods
The periodic table organizes elements into groups and periods, providing a systematic way to understand their properties and relationships. This arrangement reveals patterns in electron configuration, chemical behavior, and physical characteristics.
Main Group Elements
Main group elements, also known as representative elements, occupy groups 1-2 and 13-18 of the periodic table. These elements have their valence electrons in s and p orbitals, determining their chemical properties. Group 1 (alkali metals) and Group 2 (alkaline earth metals) are highly reactive, while Group 18 (noble gases) are generally inert. The main group elements include metals, nonmetals, and metalloids, exhibiting a wide range of properties and applications in everyday life and industry.
Transition Metals
Transition metals occupy groups 3-12 of the periodic table, filling their d-orbitals as you move across each period. These elements display unique characteristics, such as variable oxidation states, catalytic properties, and the ability to form colored compounds. Transition metals are known for their high melting points, electrical conductivity, and ductility. Many transition metals, like iron, copper, and gold, have been crucial in human technological development and are extensively used in various industries, from construction to electronics.
Lanthanides and Actinides
Lanthanides and actinides, collectively known as f-block elements, are located at the bottom of the periodic table. Lanthanides, elements 57-71, are characterized by the filling of 4f orbitals. These elements share similar chemical properties and are often used in technology, such as in magnets and lasers. Actinides, elements 89-103, involve the filling of 5f orbitals. Many actinides are radioactive and synthetic, with applications in nuclear energy and weapons. Both lanthanides and actinides exhibit unique magnetic and spectroscopic properties, making them valuable in research and specialized applications.
Trends and Patterns in the Periodic Table
The periodic table exhibits several significant trends and patterns that provide insights into element properties and behavior. These trends are crucial for understanding chemical reactions and predicting element characteristics.
Atomic Size
Atomic size generally decreases from left to right across a period and increases from top to bottom within a group. This trend is due to the increasing number of protons in the nucleus and the addition of electron shells. For example, lithium (atomic number 3) has a larger atomic radius than fluorine (atomic number 9) in the same period, while potassium (atomic number 19) is larger than sodium (atomic number 11) in the same group.
Electronegativity
Electronegativity, the ability of an atom to attract electrons in a chemical bond, increases from left to right across a period and decreases from top to bottom within a group. Fluorine, located in the upper right corner of the periodic table, has the highest electronegativity value of 3.98 on the Pauling scale. In contrast, francium, found in the lower left corner, has the lowest electronegativity at 0.7.
Ionization Energy
Ionization energy, the amount of energy required to remove an electron from an atom, generally increases from left to right across a period and decreases from top to bottom within a group. Noble gases have the highest ionization energies due to their stable electron configurations. For instance, helium has the highest first ionization energy at 2372.3 kJ/mol, while cesium has one of the lowest at 375.7 kJ/mol. This trend is closely related to atomic size and electron shielding effects.
Practical Applications of the Periodic Table
The periodic table’s practical applications extend far beyond its role as a fundamental scientific tool. Its systematic organization and wealth of information make it invaluable in various fields, from chemistry and material science to education and research.
Chemistry and Material Science
In chemistry and material science, the periodic table is an indispensable resource. It guides the development of new materials by providing insights into element properties and potential combinations. For example, semiconductor manufacturers use the table to identify elements with suitable electron configurations for creating efficient transistors. The periodic table also aids in predicting chemical reactions, helping chemists design more effective catalysts for industrial processes. In metallurgy, it’s crucial for understanding alloy formation, as the position of elements on the table indicates their ability to form solid solutions or intermetallic compounds.
Education and Research
The periodic table serves as a cornerstone in chemistry education, providing students with a visual representation of element relationships and trends. It’s an essential tool for memorizing element properties and understanding concepts like valence electrons and atomic structure. In research, scientists use the periodic table to predict the properties of newly synthesized elements and to guide their search for undiscovered ones. It’s particularly valuable in fields like nuclear physics, where researchers use the table to study isotope stability and radioactive decay patterns. The periodic table also facilitates interdisciplinary research, helping biologists understand the role of trace elements in living systems and geologists interpret the composition of minerals and rocks.
The Future of the Periodic Table
The periodic table continues to evolve as scientists explore the boundaries of atomic structure and elemental synthesis. Here’s a glimpse into the exciting developments shaping its future.
Potential New Elements
Scientists are constantly pushing the limits of element creation, focusing on synthesizing superheavy elements beyond the current boundaries of the periodic table. These elements, with atomic numbers greater than 118, are typically unstable and exist only for fractions of a second. Research teams worldwide are working on producing elements 119 and 120, which would start the eighth row of the periodic table. The challenges in creating these elements lie in the extreme conditions required for their synthesis and the difficulty in detecting their fleeting existence. As technology advances, we may see the addition of these elements, potentially altering our understanding of atomic structure and chemical properties at the extremes of the periodic table.
Ongoing Research and Discoveries
Current research in elemental science extends beyond just creating new elements. Scientists are exploring the properties of recently discovered elements to better understand their behavior and potential applications. For example, studies on element 117, tennessine, are ongoing to determine its chemical properties and how it fits into the halogen group. Additionally, researchers are investigating the “island of stability,” a theoretical region of the periodic table where superheavy elements might have longer half-lives. This concept suggests that certain isotopes of superheavy elements could be more stable than others, potentially leading to new elements with practical applications. Advancements in particle accelerators and detection methods are crucial in these endeavors, allowing for more precise experiments and observations of short-lived elements.
The periodic table of elements stands as a cornerstone of scientific understanding. Its elegant structure reveals the intricate relationships between elements shaping our world. From predicting chemical behaviors to guiding groundbreaking research this tool continues to inspire and inform.
As we look to the future I’m excited by the ongoing discoveries in elemental science. The quest for new superheavy elements and deeper insights into existing ones promises to expand our knowledge even further. The periodic table remains an indispensable guide illuminating the path of scientific progress.